The 2020 Nobel Prize in Chemistry
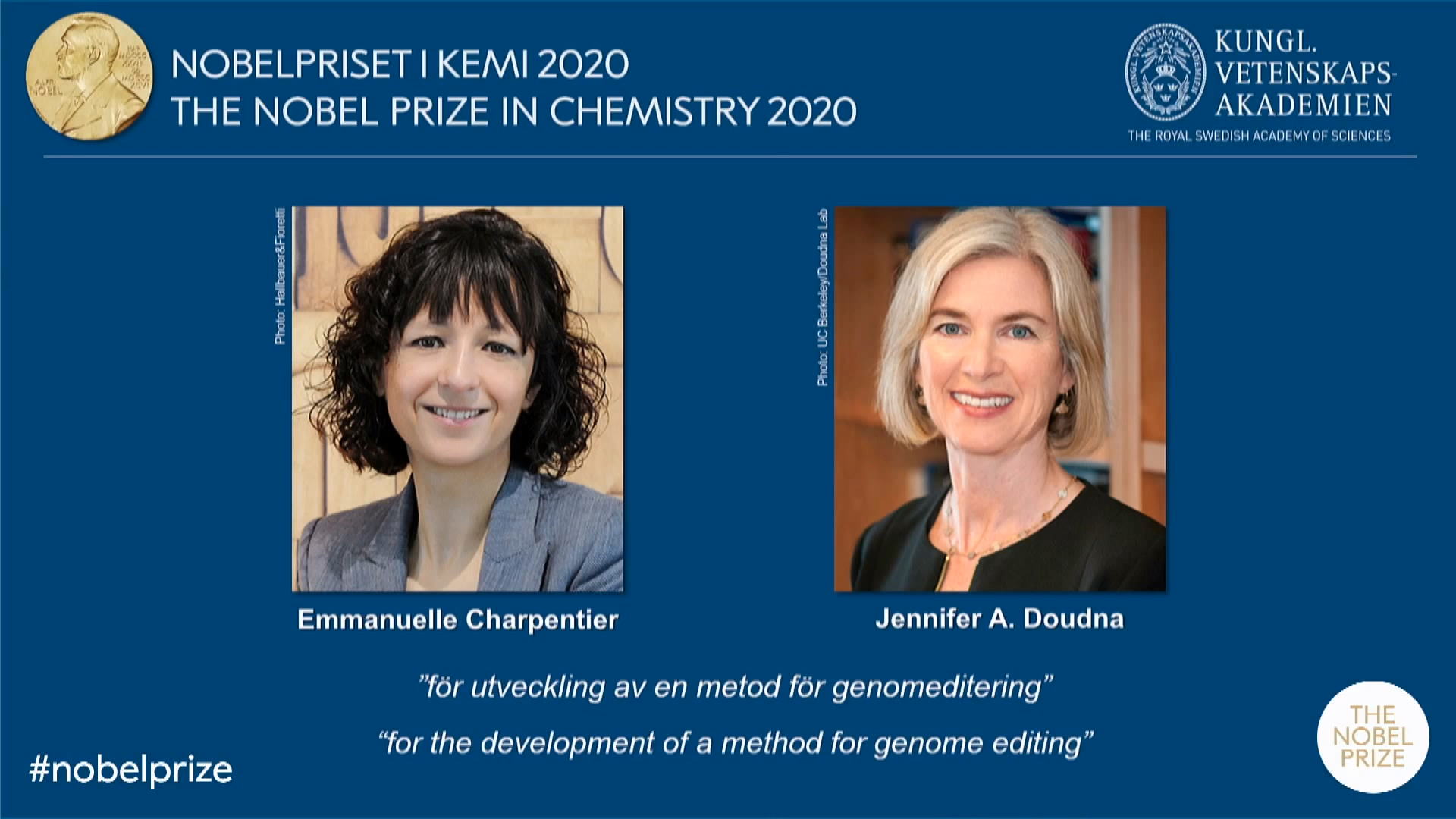
The 2020 Nobel Prize in Chemistry, which was announced on 7th October, has been awarded to Emmanuelle Charpentier and Jennifer A. Doudna “for the development of a method for genome editing.” Jennifer A. Doudna is a Howard Hughes Medical Institute Investigator and the Li Ka Shing Chancellor’s Chair in Biomedical and Health Sciences and Professor of Biochemistry, Biophysics and Structural Biology at the University of California, Berkeley (UCB). Emmanuelle Charpentier is the Founding, Scientific and Managing Director of the Max Planck Unit for the Science of Pathogens (MPG), Berlin.
Click here for the press release.
1. CRISPR
One of gene technology’s sharpest tools are the CRISPR/Cas9 genetic scissors. CRISPR, shorthand for clustered regularly interspaced short palindromic repeats, had been in study for nearly two decades but as the aughts of this millennium neared to an end, things started getting exciting. An important step towards understanding the function of CRISPR came with the identification of CRISPR-associated (cas) genes, a group of genes only present in CRISPR-containing prokaryotes and always located adjacent to CRISPRs.
In 2005, researchers noted that the unique CRISPR sequences were derived from transmissible genetic elements, such as bacteriophages and plasmids. Prokaryotes carrying these specific sequences appeared protected from infection. This was because plasmids or viruses containing a sequence matching a spacer (named protospacers) were usually absent in the prokaryote carrying the spacer. These correlative findings suggested a function for CRISPRs in prokaryotic defence against invading foreign DNA. The spacer sequences were described as a ‘memory of past “genetic aggressions.”
It had been previously shown that CRISPRs were transcribed into long RNA molecules (pre-crRNA), which were subsequently processed by cleavage within the repeat sequences to yield small CRISPR-RNAs (crRNAs). The identified cas genes encoded proteins with helicase and nuclease motifs suggesting a role in DNA metabolism or gene expression .
The association with CRISPR was used as a defining characteristic and over the coming years a number of Cas protein subfamilies were described and their roles better understood. In the Class 1 systems, specialised Cas proteins assemble into a large CRISPRassociated complex for antiviral defence (Cascade). The Class 2 systems are simpler and contain a single multidomain crRNA-binding protein (e.g. Cas9) that contains all the activities necessary for interference.
The identification of CRISPR-Cas9 as a tool for genomic editing came from studies of the Class 2 CRISPR-Cas system in the bacteria Streptococcus thermophilus, and the related human pathogen Streptococcus pyogenes. This system contains four cas genes, three of which (cas1, cas2, csn2) are involved in spacer acquisition, whereas the fourth, cas9, is needed for interference. To further define the elements required for immunity, the S. thermophilus CRISPR-Cas system was introduced into E. coli, where it provided heterologous protection against infection with phages and plasmids. Using this experimental model, parts of the system were inactivated to define the components required for protection. This clearly demonstrated that the Cas9 protein alone was sufficient for the CRISPR-encoded interference step, and that two nuclease domains present in the protein, HNH and RuvC, were both required for this effect.
2. Emmanuelle Charpentier
In 2011, Emmanuelle Charpentier, then at the Max F. Perutz Laboratories at the University of Vienna and at the Laboratory for Molecular Infection Medicine Sweden (MIMS) at Umeå University, and her lab published a seminal article in Nature reporting on the mechanisms of crRNA maturation in S. pyogenes and using differential RNA sequencing to characterize small, noncoding RNA molecules, they identified an active CRISPR locus, based on expression of pre-crRNA and mature crRNA molecules.
Unexpectedly, the sequencing efforts also identified an abundant RNA species transcribed from a region upstream of the CRISPR locus, on the opposite strand of the CRISPR array. The transcript was denoted trans-encoded small RNA (tracrRNA) and contained a stretch of 25 nucleotides (nt) with almost perfect complementarity (1-nt mismatch) to the repeat regions of the CRISPR locus, thus predicting base pairing with pre-crRNA. The RNA duplex region that would form included processing sites for both pre-crRNA and tracrRNA, which immediately suggested that the two RNAs could be co-processed upon pairin. In support of the proposed idea, deletion of the tracrRNA locus prevented pre-crRNA processing and vice versa.
Charpentier’s team also noted that a co-processed duplex involving tracrRNA and pre-crRNA would have short 3′ overhangs, similar to those produced by the endoribonuclease RNase III, and they went on to demonstrate that this enzyme could process a heteroduplex formed between tracrRNA and pre-crRNA in vitro and was required for tracrRNA and pre-crRNA processing in vivo. They also found that processing also involved the Cas9 protein, since deletion of the cas9 gene in bacteria impaired both tracrRNA and precrRNA processing, and their findings suggested that the Cas9 protein acts as a molecular anchor that facilitates base pairing between tracrRNA and pre-crRNA, which in turn allows recognition and cleavage by the host RNase III protein.
3. Collaboration with Jennifer Doudna
In 2012 Charpentier initiated a prolific collaboration with Jennifer Doudna, an experienced biochemist at the University of California, Berkeley with vast knowledge of RNA. Together, they succeeded in recreating the bacteria’s genetic scissors in a test tube and simplifying the scissors’ molecular components so they were easier to use. In a landmark paper in the journal Science, Charpentier and Doudna reported “that the Cas9 endonuclease can be programmed with guide RNA engineered as a single transcript to cleave any double-stranded DNA sequence.” It was this discovery that led to widespread applications of the CRISPR-Cas9 system as a powerful and versatile tool in genome editing!
In their study, Charpentier, Doudna and colleagues also worked to delineate the regions of tracrRNA and crRNA that are absolutely required for Cas9-catalysed cleavage of target DNA. This led to the identification of an activating domain in tracrRNA and the realisation that a “seed region” of ∼10 nt in the PAM-proximal region of the target strand was especially important for target recognition.
Based on their in vitro biochemical analysis, they hypothesized that the structural features in the two RNA molecules required for Cas9-catalysed DNA cleavage could be captured in a single RNA molecule. In a crucial experiment, they demonstrated that this was indeed possible: the RNA components (crRNA and tracrRNA) of the Cas9 complex could be fused together to form an active, chimeric single-guide RNA molecule (sgRNA). Furthermore, Charpentier and Doudna demonstrated that the sequence of the chimeric sgRNA could be changed so that CRISPR-Cas9 would target DNA sequences of interest, with the only constraint being the presence of a PAM sequence adjacent to the targeted DNA. They had thus created a simple two-component endonuclease, containing sgRNA and Cas9, that could be programmed to cleave DNA sequences at will.
4. Consequences
By introducing a vector encoding the Cas9 nuclease and an engineered sgRNA, scientists were now able to make precise single-base-pair changes or larger insertions. Coupled with the availability of genome sequences for a growing number of organisms, the technology allowed researchers to explore these genomes to find out what genes do, move mutations that are identified as associated with disease into systems where they can be studied and tested for treatment, or where they can be tested in combinations with other mutations.
5. Concluding Remarks
This was a classic example of a Nobel Prize with the question of when not if. Since the discovery of the CRISPR-Cas9 mechanism by Doudna and Charpentier, the molecular scissors have taken laboratories around the world by storm. It was a matter of time before they were recognised for their pioneering contributions. The CRISPR-Cas9 gene-editing technology has applications across diverse fields of science, including human and veterinary medicine, agriculture, and biotechnology. The revolutionary technology has enabled efficiently targeted modification of crops and is currently being developed to treat and cure genetic diseases. For instance, by modifying hematopoietic stem cells to treat sickle cell disease and β-thalassemia. It is also used in formulating quick accurate tests for COVID19.
While its broad applicability, versatility and ease of use have led to rapid adoption by the scientific community, it also brings in huge questions of ethics as the deployment of CRISPR in the human germline remains fraught with risks. CRISPR is also a hallmark of the importance of basic science research, the driving force behind which is, above all, an unquenchable curiosity about the world and its complex relationships.
Some might wonder about the logic of awarding a Chemistry Nobel to what seems to be an important biological breakthrough, but it’s important to note that bond-breaking and bond-making lie at the heart of the chemical sciences. We’re finally at a stage when we can use the full set of physical and chemical tools at our disposal to tackle the big biological questions of our time. Chemistry through its use of structure determination techniques and small molecules will further allow us to interrogate the function and find out the structure of increasingly complicated biological assemblies and enable us to uncover life’s deepest secrets.
While this Nobel Prize was undoubtedly a well deserved one, it also serves as an illustration of the fallacy of prizes, highlighting a few and hiding the contribution of many. There would be literally no CRISPR without Francisco Mojica, the unheralded microbiologist, at the University of Alicante in Spain. In 1993, he identified peculiar repetitive DNA sequences in the genome of the archaeal microbe Haloferax mediterranei, figured out that they were a bacterial immune system!, and named them as CRISPR!! Then there are the myriad contributions of folks like Ishino, Zhang, Horvath, Barrangou, and Šikšnys which established CRISPR on a firm footing. Its high time the Nobel committee reflects on the serious shortcomings of its obscure rules that have no place in today’s world of highly collaborative and enterprise-driven science and only seeks to attenuate existing biases. In a way today’s prize to two women seeks to serve as solace for the committee’s terrible record with women and one sincerely hopes that, in the heartwarming words of today’s laureate, Emmanuelle Charpentier, “This will provide a positive message to the young girls who would like to follow the path of science, and to show them that women in science can also have an impact through the research that they are performing!!”